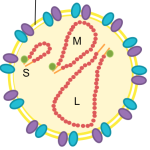
As we’re now only one week from the International Meeting on Arboviruses and their Vectors, being held in Glasgow with the Society for General Microbiology (#IMAV15), we’d like to present to you the third in a series of posts about arboviruses. This post, written by Veronica Rezelj, PhD student (@verorezelj), focuses on arboviruses themselves, their virology and how by understanding how they work, we can make safe and effective vaccines against them.
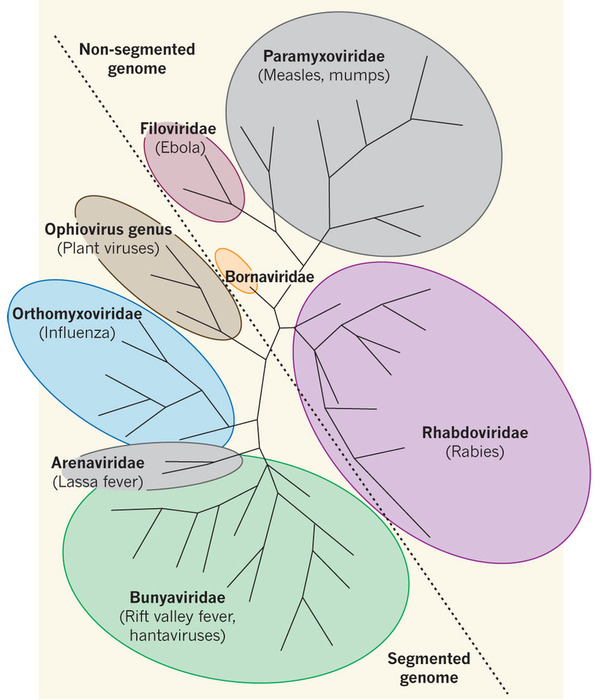
The members of the Bunyaviridae family are collectively called bunyaviruses, and they make up the largest family of viruses with an RNA genome. As explained in our previous post on arboviruses, these are almost exclusively RNA viruses, which makes the Bunyaviridae family at the present time the largest group of arboviruses, with over 350 members distributed worldwide (hence the title referring to ‘We are family’ by Sister Sledge).
The family comprises five genera: Tospovirus, Hantavirus, Nairovirus, Orthobunyavirus and Phlebovirus. With the exception of Hantaviruses and Tospoviruses, all other bunyaviruses are arboviruses whose natural lifecycle involves replication in arthropods (mosquitoes, sandflies, midges and ticks) and vertebrates. And although Tospoviruses are also transmitted by arthropods (thrips species), their natural life cycle doesn’t involve any vertebrate replication. Instead, they are plant pathogens that cause significant worldwide crop losses. The ‘odd-one-out’ are hantaviruses, which can infect vertebrates, however, they are rodent-borne viruses (roboviruses) that are transmitted to humans through infected excretions and secretions.
“Bunyaviruses make up the largest family of RNA viruses, with over 350 members distributed worldwide.”
As research on arboviruses has not been prioritised in the past, relatively little is known about the strategy for transmission and infection of many bunyaviruses. However, it became evident in the last few decades that arthropod-transmitted members of the Bunyaviridae are important pathogens in vertebrates (including humans), with a number of emerging viruses that can pose a veterinary and public health threat.
What do bunyaviruses look like?
Bunyaviruses are spherical, enveloped particles that are about 100nm in diameter (to give you an idea, this is 0.0001 millimeter or 1/10,000th of one millimeter). Their genome is single-stranded RNA, with three segments conveniently named small (S), medium (M) and large (L).
Schematic diagram of a bunyavirus virion. Credit: Veronica Rezelj
Compared to other viruses, their structure is quite simple. They have an envelope, which is composed of a lipid bilayer embedded by two external glycoproteins called Gn and Gc (encoded by the M segment). The 3 genome segments of the virion are found in the form of ribonuclear protein complexes (RNPs) in association with nucleocapsid proteins that wrap the RNA (encoded by the S segment) and RNA-dependent RNA polymerase (encoded by the L segment).
In addition to these 4 structural proteins, their genomes may encode for one or two additional non-structural proteins, named NSm (encoded by the M segment) and NSs (encoded by the S segment). These proteins have evolved various functions that help at different stages of the virus life cycle, either in its vertebrate host or its arthropod vector. In some cases, the function of the non-structural proteins is not entirely clear.
Can bunyaviruses make you sick?
As the Bunyaviridae family is so large, it is hard to generalise whether or not they can make you sick. There are many bunyaviruses that do not cause disease in humans, but there are also some that can. And within those that can, there is a spectrum of diseases that they can cause, ranging from mild self-limited febrile illnesses to haemorrhagic fevers, which can be fatal to man. Moreover, there are also some viruses that can cause disease in other vertebrates and not humans. Livestock is particularly of interest, as arboviral disease can have huge economic impacts. Below, I list a few bunyaviruses that can cause disease, and give some information on their mode of transmission, vector, and the type of disease caused.
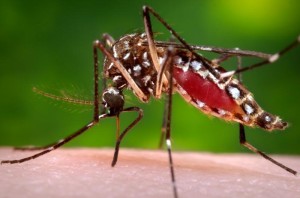
Rift Valley Fever virus (RVFV) is a phlebovirus that can cause devastating disease, inducing abortion in ruminants in Africa, and occasionally infecting humans. In its mild form, RVFV infection can result in a febrile illness with muscle and joint pain. In a minority of cases, infection can lead to ocular disease, meningoencephalitis, or hemorragic fever. Human cases are usually restricted to people who come into close contact with blood from livestock, such as veterinarians, butchers, etc. Among animals, RVFV is spread through biting of infected mosquitoes, primarily Aedes mosquitoes. Dried eggs of Aedes mosquitoes allow the sustainable maintainance of RVFV in nature for years in dry environments. Interestingly, the virus can exist for a prolongued period of time in a silent enzootic cycle, and then suddenly heavy rainfall can trigger an epizootic cycle in sheep, cattle and other ruminants, as a humid environment allows the dried eggs to hatch, and the population of mosquitoes that were infected transovarially increases.
Schmallenberg virus (SBV) is transmitted by biting midges (Culicoides). It is an orthobunyavirus that was discovered in 2011 in Germany and was later on isolated in many other European countries. Although it poses little risk to humans, adult ruminants show mild disease with fever, reduced milk yield and sometimes diarrhoea. Depending on the stage of gestation at which infection occurs, SBV can also cause abortions, stillbirths, and malformation in newborn animals.
“As research on arboviruses has not been prioritised in the past, relatively little is known about the strategy for transmission and infection of many bunyaviruses.”
Crimean Congo Hemorragic Fever virus (CCHFV) belongs to the genus Nairovirus and is the causative agent of Crimean Congo Hemorragic Fever (CCHF), which has worldwide clinical relevance. The virus exists in an enzootic cycle between vertebrates and ticks. It can infect cattle, sheep, goats and birds (which are thought to disseminate the virus by carrying infected ticks). In humans, it can cause outbreaks with mortality rates of up to 40%. The disease is characterised by sudden fever, muscle pain, dizziness, photophobia, sleepiness, nausea and vomiting. As the illness progresses signs of haemorrhage appear, with bruising, bleeding and mental confusion.
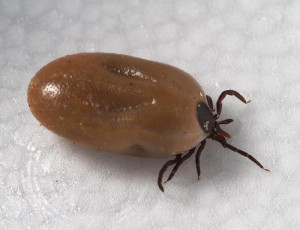
Severe Fever with Thrombocytopenia Syndrome Virus (SFTSV) This virus (which has the longest name in the Bunyavirus family) was only recently discovered. Between 2007 and 2010 there was cases of an unknown infectious disease reported in the Henan and Hubei Provinces of China, with patients presenting gastrointestinal symptoms, joint pain, thrombocytopenia and some haemorrhagic manifestations. The resulting fatality rate was of 12–30%. The causative agent of this disease was found to be SFTSV, a tick-borne phlebovirus. The virus quickly spread within China with over 570 confirmed cases and 59 deaths in 13 provinces. It was later isolated also in Japan and South Korea.
LaCrosse Encephalitis virus (LACV) is an important pathogen in North America transmitted to humans through the bite of infected mosquitoes. Symptoms of LACV infection include fever, headache, nausea, vomiting and tiredness. In more severe cases of the disease (and more commonly in children and young adults) the infection can result in encephalitis, with seizures, coma, paralysis and neurological sequelae – about 80-100 such cases are reported each year in the US.
Could climate change result in increased bunyavirus-mediated disease?
In 2008 the Wildlife Coservation Society published a report listing twelve pathogens that could spread into new regions as a result of climate change. Listed in this report is RVFV. It is believed that climate change could have an effect in the geographic distribution of different vectors of arboviral disease. In the case of bunyaviruses, this would involve mosquitoes, sandflies, midges and ticks. Although we tend to think that increased contact with vectors is the major factor driving the emergence of diseases transmitted by arthropods, the view that, in the future climate change will drive the emergence of infectious disease outbreaks is highly simplistic, because there is a far more complex interplay of factors interacting with each other that must be considered when predicting an outbreak (host, pathogen, vector and environmental factors.). However, it certainly is one factor that could contribute to disease emergence.
“The view that, in the future climate change will drive the emergence of infectious disease outbreaks is highly simplistic, because there is a far more complex interplay of factors interacting that must be considered when predicting an outbreak. However, it is certainly one factor that could contribute to disease emergence and re-emergence.“
Take RVFV for example. RVFV was first identified in Kenya in 1930, later spread outside its endemic region within Africa and continued to disperse to be identified in over 30 countries. In the year 2000, RVFV marked its first outbreak identified outside Africa when it spread to the Arabian Peninsula. Inevitably this leads to the question whether the virus can further disperse to the EU. A review in 2013 argued that although conditions have not coincided yet sufficiently for RVFV disease to spread to the US and EU, the risk of its sporadic introduction is relatively high. Due to the fact that outbreaks of RVFV occur after heavy rainfall when Aedes eggs hatch and the population of infected mosquitoes increases (as explained earlier), climatic conditions seem to be an important governant of RVFV epidemiology. Although not the sole determinant, in theory, the appropriate conditions could be generated as a result of climate change in southern Europe and the US, which could enable the emergence of the virus in these regions.
Do we have vaccines against bunyaviruses?
There is no such thing as a vaccine against all bunyaviruses, and in fact only a few bunyaviruses have been the focus for vaccine development. With the intention of breaking the chain of transmission of a virus that is difficult to control, many efforts have been made to develop a RVFV vaccine, as it poses a public health and economic burden and because it is a concern for biosecurity. Modified live attenuated and formalin-inactivated vaccines have been developed for veterinary use. And although the inactivated vaccines work well, there is a requirement for repeated immunizations to maintain immunity against RVFV, which is why live attenuated vaccines were developed, as they are highly immunogenic and can provide long-term immunity. Other ways to control RVFV involves controlling its mosquito vector through larviciding (destroying mosquitoes at their larval stage, before they reach maturity) at breeding sites.
Similarly, although an inactivated vaccine was developed for immunisation of cattle and sheep against SBV, there are currently no live-attenuated vaccines licensed for use for SBV. However, the simultaneous development of a reverse genetics system by two groups from the CVR focusing on arboviruses (the Elliott group, which was based at St Andrews University at the time, and the Palmarini group), provided the basis for the creation of attenuated strains of SBV that could act as candidate vaccines in the future, which are currently being tested.
“A pivotal strategy that certainly plays a role in controlling and reducing infection is raising awareness of risk factors, modes of transmission of the viruses and educating about ways of reducing exposure to their arthropod vectors”.
In the case of CCHF, the absence of an approved vaccine means that general supportive care and in some cases ribavirin (a nucleoside inhibitor that stops viral RNA synthesis) therapy are the main approach to treat CCHF patients. Because outcomes of CCHF infection can be severe, care of infected patients is similar to the one developed by WHO for Ebola or Marburg hemorrhagic fever. Fortunately last year, a novel recombinant vaccine carrying CCHFV glycoproteins (which are the target for neutralising antibodies) was developed, and proved to be 100% effective in a mouse model, protecting all mice from lethal disease, giving hope for a future effective vaccine that would protect people from such a nasty disease.
In contrast to RVFV and SBV, no vaccines are currently available for LACV or SFTSV, all human pathogens. For SFTSV, however, recent advances in its reverse genetics system by the Elliott group at the CVR could facilitate the engineering of safe and effective live-attenuated vaccines in the future.
What else can we do to stop them?
It is important to emphasize that although arbovirus control seems to be inherently complex due to their ecology and biology –after all, they are able to survive in and fight two completely different systems!- a pivotal strategy that certainly plays a role in controlling and reducing infection in people is raising awareness of risk factors, modes of transmission of the viruses and educating about ways of reducing exposure to their arthropod vectors.
*********************************************************************************************************************
The MRC-University of Glasgow Centre for Virus Research will host the 2015 International Meeting on Arboviruses and their Vectors (#IMAV15), as a Society for General Microbiology-sponsored ‘focus meeting’. #IMAV15 will be held on Monday 7th and Tuesday 8th September 2015 in Glasgow and confirmed speakers include Marco Vignuzzi (Pasteur Institute, France), Michael Diamond (Washington University in St Louis, USA), Lisa Fong Poh Ng (A*Star, Singapore) and Zoe Curtis (Oxitec, UK).
The CVR has a broad, collaborative programme on arboviruses as part of its ‘Emerging & zoonotic viral infections’ theme. In particular, the CVR investigates their molecular virology, virus-host interactions, vaccine development and vector biology across the different programme member labs of Alain Kohl, Esther Schnettler, Emilie Pondeville, Margus Varjak, Richard Elliott and Massimo Palmarini. This strategy allows us to be prepared for future, often unpredictable, problems with arboviruses, but also work on ongoing issues.
*********************************************************************************************************************